Turning exotic materials
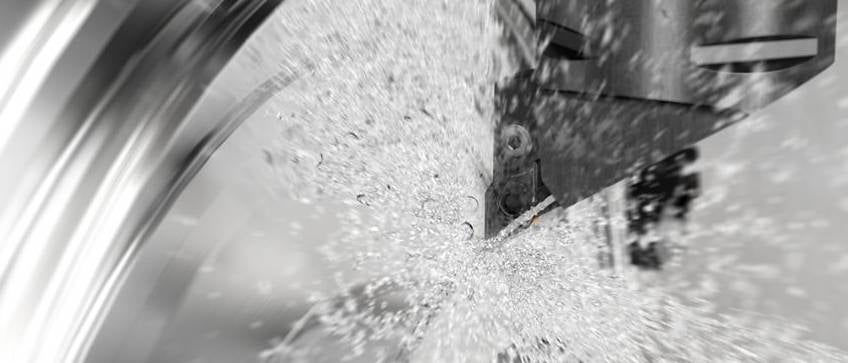
Heat-resistant superalloys and titanium alloys are demanding to machine. They are like no other metals and, as such, exotic. They have superb properties, such as strength-to-weight ratio, strength and hardness retention at high temperatures and excellent corrosion resistance.
However, as the materials are also demanding to machine, they need “exotic” solutions. These have to be well planned with dedicated tools and carefully chosen methods and tool-paths, in order to guarantee efficiency, security and good results.
This overview looks at machining demands and developments that have elevated performance and process security in turning.
Unique component properties, but demanding to machine
The ability to machine a component material is determined by several factors which influence and determine requirements and outcomes in metal cutting operations. In a very broad sense, it is the ability of the component material to be cut in relation to the tendency for tool wear to be generated and how chips are formed. With the difference that, on most levels, these exotic materials have poor machinability. They are considered demanding to cut, but not difficult if approached in the right way.
The more exotic of the relatively common component materials are classified under the ISO S group: heat-resistant super alloys (HRSA) and titanium alloys. For machining, these can all be split into several sub-groups, depending on composition, condition and properties. The chemical nature and metallurgical composition of an S-classified alloy will determine the physical properties and consequently machinability. Chip control is generally demanding due to segmentation of chips, and it is not unusual for the specific cutting force to be twice that of steel (i.e. the direct measurement of how hard it is to cut a material, which determines the cutting force and power needed).
The main reason that HRSA materials are demanding to cut is because they retain high strength at high temperatures. They do not soften and flow in the way most other materials do, and they also work-harden readily. High mechanical load and considerable heat are concentrated on the cutting edge. Nickel-, iron- or cobalt-based alloys are sub-groups of HRSA, having unique capabilities for component use, mainly in aerospace-, energy- and medical industries, as their advantageous properties do not change much until they approach their melting point. They are also very anti-corrosive. However, from a machinability point of view they need a capable machine, rigid set-up conditions, dedicated insert grades and geometries, optimized coolant application and, last but not least, the right machining method and tool approach. Certainly more planning is needed, requiring more work at the front end of manufacturing.
Titanium alloys are also divided into sub-groups with varying machinability grading. Generally, machinability is rated as various degrees of poor (toughness), which places very special demands on tools and methods. Demands are set by low thermal conductivity, high strength at high temperatures, highly-sheared, thin chips with a tendency for galling, creating a narrow contact area on the insert rake face, and high cutting forces concentrated close to the cutting edge. Chips can have cyclic formation, leading to variable cutting forces, and some alloys have a relatively high level of carbides making the material extra-abrasive. Excessive cutting speeds can give rise to a chemical reaction between chip and tool material, resulting in sudden chipping/fracture of the cutting edge and also material smearing/welding on cutting edges. Some alloys also work-harden readily, giving rise to diffusion-type wear, which leads to excessive burr formation. This can also make the following operations difficult.
Exotic, yes, but the window for successful machining of many HRSAs and titanium alloys is relatively small.
When turning these materials…
… a successful outcome hinges on balancing the combined effect of the material and the application factors. There are a few basic rules-of-thumb which contribute hugely to good results:
- pre-plan a sound machining strategy which is as detailed as possible,
- establish the best tool approach,
- establish the best tool-paths and use very stable tool clamping,
- use the best new, dedicated cutting tool technology,
- apply qualified cutting data to establish process security and productivity,
- use spiral cutting length calculation for predicting cuts,
- apply coolant correctly — modern high pressure solutions,
- make use of specialist recommendations and support.
The machining process should always be carefully planned because of the critical, decisive factors involved, such as consideration to the state/condition of the workpiece material. Cast, forged, bar-stock, heat treatment, solution treatment and aging considerably affect the component in ways that should influence the selection of tools and methods. The workpiece surface condition and hardness vary and affect machining. The turning strategy should also include the demands imposed by the design features of the components to be machined, as well as the various stages of machining, in terms of roughing (first stage), semi-finishing (intermediate stage) and finishing (last stage). Complex features and surface integrity are common issues.
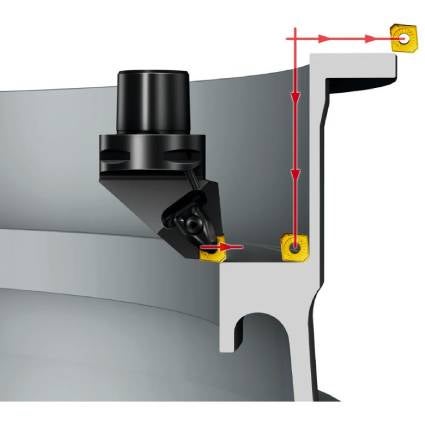
When planning turning of exotic materials, the component design, material and condition will form the basis. If applicable, establish how first-, intermediate- and last-stage machining should be carried out, in relation to the material condition and the quality demands. Planning tool paths and using feed reduction when programming will determine the extent of wear, tool type, cycle times and security.
The cutting action in these materials…
… is to a great extent affected by the cutting edge's approach to the workpiece. The entering angle of the cutting edge, in combination with the insert geometry, determines the performance, tool life, security and results. Insert shapes often have to be chosen in relation to the required cut. However, the fact that using a small entering angle contributes to performance and tool life should always be a key consideration for the application.
The choice of insert grade needs to be made partly in relation to the entering angle. Among other things, this angle influences the type of wear that forms a notch on the cutting edge, the size of which affects results and can cause premature tool failure. Getting the approach part of the application right also means that an insert grade capable of higher productivity can be chosen, while also guaranteeing a long tool life and security.

The entering angle of the cutting tool affects the chip thickness, the feed rate, the cutting forces and the type of cuts that are possible. With exotics, the choice of angle has direct consequences for the productivity and process reliability, influencing the insert shape and the nose radius, and how well the tool material can be utilized. Round inserts and Xcel-type inserts are normally the best choices.
The choice of tool material (insert grade) should be influenced by the type of turning operation involved (roughing, semi-finishing or finishing), as well as the workpiece condition and the type of cut. Due to the hardness of these materials, plastic deformation of the cutting edge should always be considered as the primary risk factor when selecting the insert grade (notch wear, as mentioned, is mainly affected by the entering angle and depth of cut). Insert grade selection should also be combined with the insert shape, since this is a strength issue to be assessed with the approach, chip load and whether the cut is continuous or interrupted. Dedicated grades are consequently vital for HRSA machining.
A high degree of insert hot hardness, the right level of insert toughness and sufficient adhesion of the insert coating are the primary requirements. Positive cutting geometry, a sharp cutting edge, a strong edge and a comparatively open chip breaker should characterize the indexable insert for these materials.
Establishing the most suitable cutting data is vital to success in these materials. Cutting speed is limited to the combination of speed, feed and depth of cut, and can be optimized to provide high levels of productivity, security and quality. The cutting speed is related to heat generation and how this affects the insert; it needs to be high enough for the chip to have sufficient plasticity but not so high as to unbalance the tool material. Speeds are usually in the region of 130 to 260 sfm (40 to 80 m/min) with dedicated cemented carbide inserts and 490 to 1310 sfm (150 to 400 m/min) with ceramic inserts.
The feed rate is the main factor that affects the cutting time and the chip thickness. In exotics, this needs to be more carefully balanced as limits are relatively tight: in roughing, the chip must be maximized but not so as to overload the edge, while in finishing, the chip needs to be thick enough to prevent excessive heat and work hardening.
The depth of cut often affects the approach of the edge and consequently must be below a certain value. For example, when using round inserts in HRSA, the depth of cut should not exceed 15% of the insert diameter. The depth of cut must also be programmed carefully when profiling. Recesses or shoulders are integrated to avoid exceeding the suitable arc of cutting edge engagement.
In terms of tool life, it is important to work extensively with the spiral cutting length (SCL). Establishing this correctly means that machine stoppages for insert indexing can be predicted and programmed, and that passes with a tool used at the right speed can be completed without the cutting edge becoming incapable of maintaining the required surface quality.
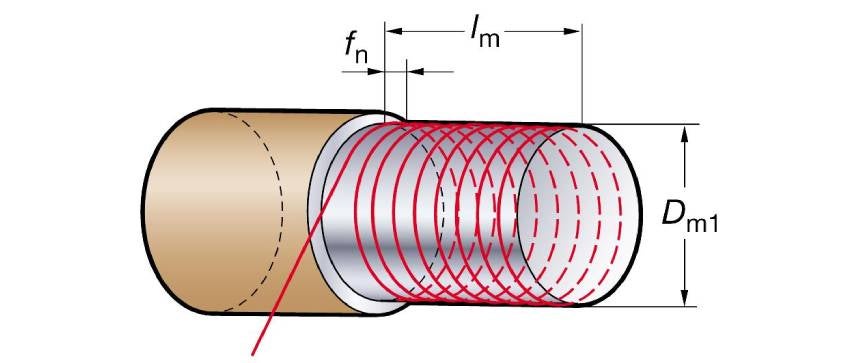
Using the spiral cutting length calculations will help in predicting the time or cutting length, so as to reduce the machining time and improve the surface finish in demanding exotic materials. The machine stoppages for insert changing are then planned with spiral length cutting to better suit the operation and cutting data to optimize tool life and machine utilization.
Tool wear is greatly affected…
…by the high cutting forces in combination with higher cutting edge temperatures, with a tendency for certain types of cutting edge wear to develop. The main types are notch wear (the mechanical wear type where the depth of cut sets the workpiece material line); plastic deformation of the cutting edge — a consequence of the combined high temperature and pressure, and thirdly abrasive wear caused mainly by the harder materials. Another type is top-slice wear, which develops on ceramic inserts where layers of the top of the cutting edge are sliced off. The approach of the cutting edge plays a major role.
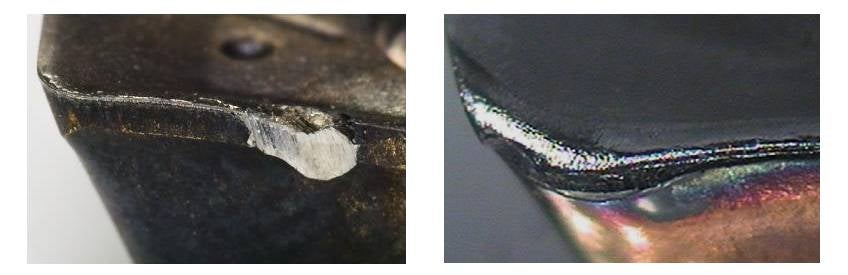
The insert grade selection is not as directly related to roughing and finishing of exotics as it is in other materials. Instead, grade selection is more of an optimization factor – a little bit more complex, dependent upon the shape of the insert and the approach of the cutting edge and the type of operation. Destructive wear patterns, such as the notch wear and plastic deformation shown, must be contained mainly by a combination of suitable tools, cutting data and method. Cemented carbide inserts along with modern sialon ceramics and, to some extent whiskered ceramics, are often the most suitable and broadly applicable choice.
The most suitable entering angle for turning exotics is a small angle of 45 degrees or less. The worst entering angle is 90/0 degrees, or when the depth of cut is larger than the nose radius of the insert. A large/small angle means a thin chip and higher feeds.
For HRSA turning, the tool's entering angle will determine whether a PVD- or CVD-coated insert is the most suitable. This is to protect against thermal effects. PVD is suitable for a 90/0 degree entering angle and CVD for 45 degrees. Titanium-turning, on the other hand, does not benefit from insert coating. In this case, it is more important to ensure the most suitable cutting edge condition, for example, round inserts for finishing. New families of PVD inserts offer high hardness and good resistance to edge deformation and thermal shocks. Combined with sharp edges, they can also excel in roughing to finishing operations with smearing materials.
The right insert shape…
…is an important application factor when machining these materials. The round (R-shape) has become one of the main recommendations for these materials. The round insert offers strength for a sharp, positive cutting edge; a chip thickness that varies along a long cutting edge allowing high feed rates; a large insert radius, which does not restrict the feed rate because of the surface finish it creates. The round insert also offers the programming flexibility to perform profiling and pocketing operations required by many component shapes.
A square insert (S-shape) is in some cases the most suitable for first stage machining, with its capacity for roughing cuts in various directions with a 45-degree angle. The rhomboid insert (C-shape) has built-in flexibility in terms of tool paths and, when extended to form an Xcel-type insert, provides increased tool accessibility into corners, shoulders and recesses. This combination of the insert shape and 45-degree entering angle also reduces radial cutting forces, guarantees constant chip thickness and reduces notch wear. The result is higher productivity, longer tool life and better security.
Program optimization…
…is important, and below are a few recommendations for improved performance, especially when using round inserts, which are basically suitable for exotic materials:
- avoid plunging into cuts and softening impacts; if these cuts are necessary, halve the feed rate,
- when turning to a shoulder, the feed should also be reduced by half or the tool should roll up to the shoulder where the programmed radius is the same as the insert diameter. (Guidelines are for the minimum programmed radius to be 25% of the insert diameter and the component radius 75% of the insert diameter. The tool center feed is for the programmed radius.),
- for roughing with round inserts, ensure that the programmed radius equals the insert diameter, and for finishing, make sure the programmed radius is larger than the insert diameter,
- consider alternative tool paths, multiple passes and machining in both directions to fully utilize inserts,
- protect ceramic inserts by pre-chamfering the workpiece and feed into the chamfer,
- maintain a satisfactory entering angle balance to the arc of insert-engagement throughout machining. Angle limitation is essential for good performance, and the use of round inserts is an important booster to realize available potential with modern insert grades,
- avoid any wrap-around effects when profiling or plunging so as not to overload the insert; use alternative tool paths or smaller insert diameter,
- consider trochoidal turning, breaking the cut up into suitable smaller cuts, especially when pocketing.
What should you be looking at in terms of tool types and methods for HRSA and titanium machining? The following are some of the main aspects:
- Precision coolant should always be on the agenda. Precision jets acting behind and at the cutting edge, supplied by through-coolant tooling, offer numerous benefits for turning, milling and drilling operations. State-of-the art nozzle technology, available as standard and engineered solutions with various pressure ranges, can be applied to all types of machinery with adequate coolant supply. Coolant pressure for new machine investments should always include coolant pressure capability of 70 bar for titanium turning to facilitate improved chip breaking, and pressure of up to 200 bar is advantageous for HRSA turning, due to harder-to-break chips.
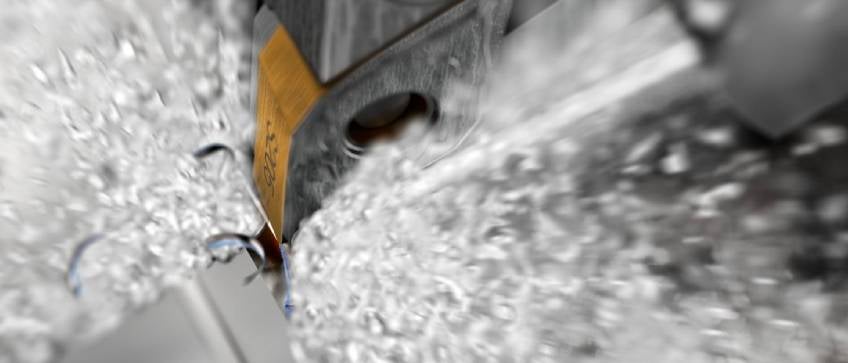
The use of coolant is a decisive application factor in machining exotics. Precision coolant application is high-tech as standard or engineered today, and so efficient as to make a clear difference to the cut, chip formation, tool life and productivity. Generally, the high temperatures generated in these materials make the cooling effect necessary, however when the coolant is applied in high volumes, at high pressure and precision-directed, in combination with dedicated inserts and through-tool coolant supply, performance and results are optimized.
- Accessibility is often an issue when turning exotics. Complex features and tool overhangs make it critical to get tooling and methods right. Look at modular systems, which include a reliable concept with tool blades presenting the cutting edges at different angles and overhangs. The range of adaptor and blade alternatives should offer the flexibility to build almost any tool from a limited standard tool inventory to suit configurations and ensure accessibility in external or internal confined spaces. Blades should include the required radial and axial clearances for reaching deep into angled grooves with precision coolant supplied through the tool to the cutting edge.
- Tool material is at the heart of any machining operation, and a critical factor for exotics. This area requires dedicated insert grades in combination with the right insert geometry, mainly in the form of the latest in cemented carbide and ceramics. Uncoated carbide grades still play an important role, but the latest developments in insert coating technology include specially coated cemented carbide inserts that have moved cutting-edge capability on by shortening the cutting time and extending tool life.
- Built-in tool-damping features in boring bars, blades and even milling cutters should be included to minimize vibration. Anti-vibration technology has taken a huge leap, and should be a natural option where tool overhangs tend to generate instability. Productivity, process security and component quality are factors directly related to the quality and availability of anti-vibration tooling. Numerous operations are impossible to perform without this facility: today, internal turning involving overhangs of up to fourteen times diameters of up to 250 mm can be performed very efficiently with a high-quality finish.